ABSTRACT
Although small interfering RNA (siRNA) holds great therapeutic promise, its delivery to the disease site remains a paramount obstacle. In this study, we tested whether brain endothelial cell-derived exosomes could deliver siRNA across the blood–brain barrier (BBB) in zebrafish. Natural exosomes were isolated from brain endothelial bEND.3 cell culture media and vascular endothelial growth factor (VEGF) siRNA was loaded in exosomes with the assistance of a transfection reagent. While fluorescence-activated cell flow cytometry and immunocytochemistry staining studies indicated that wild-type exosomes significantly increased the uptake of fluorescence-labeled siRNA in the autologous brain endothelial cells, decreased fluorescence intensity was observed in the cells treated with the tetraspanin CD63 antibody-blocked exosome-delivered formulation (p < 0.05). In the transport study, exosomes also enhanced the permeability of rhodamine 123 in a co-cultured monolayer of brain endothelial bEND.3 cell and astrocyte. Inhibition at the expression of VEGF RNA and protein levels was observed in glioblastoma-astrocytoma U-87 MG cells treated with exosome-delivered siRNAs. Imaging results showed that exosome delivered more siRNAs across the BBB in Tg(fli1:GFP) zebrafish. In a xenotransplanted brain tumor model, exosome-delivered VEGF siRNAs decreased the fluorescence intensity of labeled cancer cells in the brain of zebrafish. Brain endothelial cell-derived exosomes could be potentially used as a natural carrier for the brain delivery of exogenous siRNA.







Similar content being viewed by others
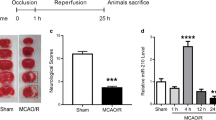
References
Borna H, Imani S, Iman M, Azimzadeh Jamalkandi S. Therapeutic face of RNAi: in vivo challenges. Expert Opin Biol Ther. 2015;15:269–85. doi:10.1517/14712598.2015.983070.
de Fougerolles A, Vornlocher HP, Maraganore J, Lieberman J. Interfering with disease: a progress report on siRNA-based therapeutics. Nat Rev Drug Discov. 2007;6(6):443–53. doi:10.1038/nrd2310.
Kubowicz P, Zelaszczyk D, Pekala E. RNAi in clinical studies. Curr Med Chem. 2013;20(14):1801–16.
Kanasty R, Dorkin JR, Vegas A, Anderson D. Delivery materials for siRNA therapeutics. Nat Mater. 2013;12(11):967–77. doi:10.1038/nmat3765.
Whitehead KA, Langer R, Anderson DG. Knocking down barriers: advances in siRNA delivery. Nat Rev Drug Discov. 2009;8(2):129–38. doi:10.1038/nrd2742.
Fan Y, Moon JJ. Nanoparticle drug delivery systems designed to improve cancer vaccines and immunotherapy. Vaccines. 2015;3(3):662–85. doi:10.3390/vaccines3030662.
Gidwani M, Singh AV. Nanoparticle enabled drug delivery across the blood brain barrier: in vivo and in vitro models, opportunities and challenges. Curr Pharm Biotechnol. 2014;14(14):1201–12.
Mishra D, Hubenak JR, Mathur AB. Nanoparticle systems as tools to improve drug delivery and therapeutic efficacy. J Biomed Mater Res A. 2013;101(12):3646–60. doi:10.1002/jbm.a.34642.
Williford JM, Wu J, Ren Y, Archang MM, Leong KW, Mao HQ. Recent advances in nanoparticle-mediated siRNA delivery. Annu Rev Biomed Eng. 2014;16:347–70. doi:10.1146/annurev-bioeng-071813-105119.
Ozpolat B, Sood AK, Lopez-Berestein G. Liposomal siRNA nanocarriers for cancer therapy. Adv Drug Deliv Rev. 2014;66:110–6. doi:10.1016/j.addr.2013.12.008.
Kooijmans SA, Vader P, van Dommelen SM, van Solinge WW, Schiffelers RM. Exosome mimetics: a novel class of drug delivery systems. Int J Nanomedicine. 2012;7:1525–41. doi:10.2147/IJN.S29661.
Lai RC, Yeo RW, Tan KH, Lim SK. Exosomes for drug delivery—a novel application for the mesenchymal stem cell. Biotechnol Adv. 2013;31(5):543–51. doi:10.1016/j.biotechadv.2012.08.008.
Lakhal S, Wood MJ. Exosome nanotechnology: an emerging paradigm shift in drug delivery: exploitation of exosome nanovesicles for systemic in vivo delivery of RNAi heralds new horizons for drug delivery across biological barriers. BioEssays. 2011;33(10):737–41. doi:10.1002/bies.201100076.
van den Boorn JG, Dassler J, Coch C, Schlee M, Hartmann G. Exosomes as nucleic acid nanocarriers. Adv Drug Deliv Rev. 2013;65(3):331–5. doi:10.1016/j.addr.2012.06.011.
Rana S, Zoller M. Exosome target cell selection and the importance of exosomal tetraspanins: a hypothesis. Biochem Soc Trans. 2011;39(2):559–62. doi:10.1042/BST0390559.
Haqqani AS, Delaney CE, Tremblay TL, Sodja C, Sandhu JK, Stanimirovic DB. Method for isolation and molecular characterization of extracellular microvesicles released from brain endothelial cells. Fluids Barriers CNS. 2013;10(1):4. doi:10.1186/2045-8118-10-4.
Alvarez-Erviti L, Seow Y, Yin H, Betts C, Lakhal S, Wood MJ. Delivery of siRNA to the mouse brain by systemic injection of targeted exosomes. Nat Biotechnol. 2011;29(4):341–5. doi:10.1038/nbt.1807.
Liu Y, Li D, Liu Z, Zhou Y, Chu D, Li X, et al. Targeted exosome-mediated delivery of opioid receptor Mu siRNA for the treatment of morphine relapse. Sci Rep. 2015;5:17543. doi:10.1038/srep17543.
Wahlgren J, Statello L, Skogberg G, Telemo E, Valadi H. Delivery of small interfering RNAs to cells via exosomes. Methods Mol Biol. 2016;1364:105–25. doi:10.1007/978-1-4939-3112-5_10.
Cooper JM, Wiklander PB, Nordin JZ, Al-Shawi R, Wood MJ, Vithlani M, et al. Systemic exosomal siRNA delivery reduced alpha-synuclein aggregates in brains of transgenic mice. Move Disord. 2014;29(12):1476–85. doi:10.1002/mds.25978.
Shtam TA, Kovalev RA, Varfolomeeva EY, Makarov EM, Kil YV, Filatov MV. Exosomes are natural carriers of exogenous siRNA to human cells in vitro. Cell Commun Signal. 2013;11:88. doi:10.1186/1478-811X-11-88.
Sun D, Zhuang X, Xiang X, Liu Y, Zhang S, Liu C, et al. A novel nanoparticle drug delivery system: the anti-inflammatory activity of curcumin is enhanced when encapsulated in exosomes. Mol Ther. 2010;18(9):1606–14. doi:10.1038/mt.2010.105..
Yang T, Martin P, Fogarty B, Brown A, Schurman K, Phipps R, et al. Exosome delivered anticancer drugs across the blood–brain barrier for brain cancer therapy in Danio rerio. Pharm Res. 2015;32(6):2003–14. doi:10.1007/s11095-014-1593-y.
Jang SC, Kim OY, Yoon CM, Choi DS, Roh TY, Park J, et al. Bioinspired exosome-mimetic nanovesicles for targeted delivery of chemotherapeutics to malignant tumors. ACS Nano. 2013;7(9):7698–710. doi:10.1021/nn402232g.
Zhuang X, Xiang X, Grizzle W, Sun D, Zhang S, Axtell RC, et al. Treatment of brain inflammatory diseases by delivering exosome encapsulated anti-inflammatory drugs from the nasal region to the brain. Mol Ther. 2011;19(10):1769–79. doi:10.1038/mt.2011.164.
Li G, Simon MJ, Cancel LM, Shi ZD, Ji X, Tarbell JM, et al. Permeability of endothelial and astrocyte cocultures: in vitro blood–brain barrier models for drug delivery studies. Ann Biomed Eng. 2010;38(8):2499–511. doi:10.1007/s10439-010-0023-5.
Bai S, Yang T, Abbruscato TJ, Ahsan F. Evaluation of human nasal RPMI 2650 cells grown at an air-liquid interface as a model for nasal drug transport studies. J Pharm Sci. 2008;97(3):1165–78. doi:10.1002/jps.21031.
Yang T, Bantegui T, Pike K, Bloom R, Phipps R, Bai S. In vitro evaluation of optimized liposomes for delivery of small interfering RNA. J Liposome Res. 2014;24:270–9. doi:10.3109/08982104.2014.907306.
Westerfield M. The zebrafish book: a guide for the laboratory use of zebrafish (Brachydanio rerio). Eugene: University of Oregon Press; 1993.
Jeong JY, Kwon HB, Ahn JC, Kang D, Kwon SH, Park JA, et al. Functional and developmental analysis of the blood–brain barrier in zebrafish. Brain Res Bull. 2008;75(5):619–28. doi:10.1016/j.brainresbull.2007.10.043.
Yang XJ, Cui W, Gu A, Xu C, Yu SC, Li TT, et al. A novel zebrafish xenotransplantation model for study of glioma stem cell invasion. PLoS One. 2013;8(4):e61801. doi:10.1371/journal.pone.0061801.
Gururangan S, Fangusaro J, Poussaint TY, McLendon RE, Onar-Thomas A, Wu S, et al. Efficacy of bevacizumab plus irinotecan in children with recurrent low-grade gliomas—a Pediatric Brain Tumor Consortium study. Neuro-Oncology. 2014;16(2):310–7. doi:10.1093/neuonc/not154.
Geldenhuys WJ, Allen DD, Bloomquist JR. Novel models for assessing blood–brain barrier drug permeation. Expert Opin Drug Metab Toxicol. 2012;8(6):647–53. doi:10.1517/17425255.2012.677433.
Gabathuler R. Approaches to transport therapeutic drugs across the blood–brain barrier to treat brain diseases. Neurobiol Dis. 2010;37(1):48–57. doi:10.1016/j.nbd.2009.07.028.
Ghosh YK, Visweswariah SS, Bhattacharya S. Nature of linkage between the cationic headgroup and cholesteryl skeleton controls gene transfection efficiency. FEBS Lett. 2000;473(3):341–4.
Forster S, Thumser AE, Hood SR, Plant N. Characterization of rhodamine-123 as a tracer dye for use in in vitro drug transport assays. PLoS One. 2012;7(3):e33253. doi:10.1371/journal.pone.0033253.
Shibuya M. VEGF-VEGFR signals in health and disease. Biomol Ther. 2014;22(1):1–9. doi:10.4062/biomolther.2013.113.
Sia D, Alsinet C, Newell P, Villanueva A. VEGF signaling in cancer treatment. Curr Pharm Des. 2014;20(17):2834–42.
Cardones AR, Banez LL. VEGF inhibitors in cancer therapy. Curr Pharm Des. 2006;12(3):387–94.
Gururangan S, Fangusaro J, Young Poussaint T, Onar-Thomas A, Gilbertson RJ, Vajapeyam S, et al. Lack of efficacy of bevacizumab + irinotecan in cases of pediatric recurrent ependymoma—a Pediatric Brain Tumor Consortium study. Neuro-Oncology. 2012;14(11):1404–12. doi:10.1093/neuonc/nos213.
Pardridge WM. Blood–brain barrier delivery. Drug Discov Today. 2007;12(1–2):54–61. doi:10.1016/j.drudis.2006.10.013.
Vlassov AV, Magdaleno S, Setterquist R, Conrad R. Exosomes: current knowledge of their composition, biological functions, and diagnostic and therapeutic potentials. Biochim Biophys Acta. 2012;1820(7):940–8. doi:10.1016/j.bbagen.2012.03.017.
Record M, Subra C, Silvente-Poirot S, Poirot M. Exosomes as intercellular signalosomes and pharmacological effectors. Biochem Pharmacol. 2011;81(10):1171–82. doi:10.1016/j.bcp.2011.02.011.
Schneider A, Simons M. Exosomes: vesicular carriers for intercellular communication in neurodegenerative disorders. Cell Tissue Res. 2013;352(1):33–47. doi:10.1007/s00441-012-1428-2.
Simons M, Raposo G. Exosomes—vesicular carriers for intercellular communication. Curr Opin Cell Biol. 2009;21(4):575–81. doi:10.1016/j.ceb.2009.03.007.
Xie X, Ross JL, Cowell JK, Teng Y. The promise of zebrafish as a chemical screening tool in cancer therapy. Future Med Chem. 2015;7(11):1395–405. doi:10.4155/fmc.15.73.
Zhang B, Xuan C, Ji Y, Zhang W, Wang D. Zebrafish xenotransplantation as a tool for in vivo cancer study. Familial Cancer. 2015;14(3):487–93. doi:10.1007/s10689-015-9802-3.
Singleman C, Holtzman NG. Growth and maturation in the zebrafish, Danio rerio: a staging tool for teaching and research. Zebrafish. 2014;11(4):396–406. doi:10.1089/zeb.2014.0976.
Asnani A, Peterson RT. The zebrafish as a tool to identify novel therapies for human cardiovascular disease. Dis Models Mech. 2014;7(7):763–7. doi:10.1242/dmm.016170.
Umans RA, Taylor MR. Zebrafish as a model to study drug transporters at the blood–brain barrier. Clin Pharmacol Ther. 2012;92(5):567–70. doi:10.1038/clpt.2012.168.
Xie J, Farage E, Sugimoto M, Anand-Apte B. A novel transgenic zebrafish model for blood–brain and blood-retinal barrier development. BMC Dev Biol. 2010;10:76. doi:10.1186/1471-213X-10-76.
ACKNOWLEDGEMENTS
Research reported in this project was supported by the Husson University Faculty Research Funds and School of Pharmacy Seed Grants.
Author information
Authors and Affiliations
Corresponding author
Rights and permissions
About this article
Cite this article
Yang, T., Fogarty, B., LaForge, B. et al. Delivery of Small Interfering RNA to Inhibit Vascular Endothelial Growth Factor in Zebrafish Using Natural Brain Endothelia Cell-Secreted Exosome Nanovesicles for the Treatment of Brain Cancer. AAPS J 19, 475–486 (2017). https://doi.org/10.1208/s12248-016-0015-y
Received:
Accepted:
Published:
Issue Date:
DOI: https://doi.org/10.1208/s12248-016-0015-y