Abstract
Purpose To assess the general feasibility of recording multifocal electroretinograms (mfERGs) with simultaneous fundus monitoring in a clinical setting.
Methods An mfERG system (RETIscan) and a confocal scanning laser ophthalmoscope (Heidelberg Retina Angiograph) were connected to record mfERGs elicited by a pseudorandom binary m-sequence stimulus generated by a 514 nm laser source. Recordings were compared to a conventional mfERG system using monitor stimulation. A total of five subjects (two normal subjects and three patients) were examined.
Results In normal volunteers records obtained by the fundus-monitored mfERG showed highest response densities in the central fundus area and a decrease of response amplitudes towards the periphery paralleling cone receptor density. However, compared to the monitor stimulation, the drop-off of amplitudes as a function of eccentricity was not as clearly defined. The responses obtained from patients with retinal diseases were diminished in the areas of retinal dysfunction.
Conclusions These preliminary findings indicate that the technique of laser stimulation in principle allows for topographic retinal recording. This method might be useful, for example if the retinal position of the stimulus array is not centred onto the fovea but deviates due to fixation problems. However, further improvement of the technique appears necessary before considering routine clinical application.
Similar content being viewed by others
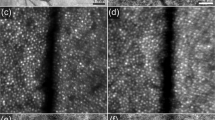
Introduction
The multifocal electroretinogram (mfERG) allows for topographic mapping of retinal function by concurrently stimulating a large number of retinal locations and extracting their local responses.1 Thus, regionally confined areas of retinal dysfunction can be detected. In conventional monitor stimulation, subjects are required to fixate a mark on the monitor during recording. It is therefore sometimes difficult to obtain reliable mfERGs from children, malingerers and patients with eccentric fixation. A normal mfERG result poses no difficulties in interpretation. Without monitoring the subject's fixation, however, it is difficult to distinguish abnormal mfERGs from records obtained with inadequate patient cooperation. Fundus monitoring during the ERG examination provides reliable information regarding the location of the stimulus on the retina. In this report we clinically assessed a method for recording mfERGs from a limited number of patients and normals with simultaneous fundus monitoring using a novel digital, confocal scanning laser ophthalmoscope.
Materials and methods
Technique
Retinal stimulation is based on a multi-input stimulation technique first applied in the Visual Evoked Response Imaging System (Electro-Diagnostic Imaging, San Mateo, USA).2,3
In this study mfERG responses were obtained with the RETIscan system (Roland Consult, Elektrophysiologische Diagnostik Systeme, Brandenburg, Germany). Elements are modified independently between two luminance levels following a short corrected binary m-sequence, and local responses of all stimulated retinal areas are extracted from the sum response.4
CSLO Stimulation
Fundus-monitored recordings were obtained by connecting the RETIscan system to a confocal scanning laser ophthalmoscope (cSLO) (Heidelberg Retina Angiograph, HRA, Heidelberg Engineering, Heidelberg, Germany) that was initially developed for fluorescein and indocyanine green angiography (Figure 1A).5 Operating on a line frequency of 125 μs in double-scan mode the fastest frame rate possible is 20 Hz. Since the software inserts an additional dark frame the effective frame rate is 10 Hz. The argon-green laser beam (3 mm illumination beam aperture) at a wavelength of 514 nm generated the stimulus array, while an infrared diode laser (830 nm) performed a concurrent scan to permit simultaneous visualization of the retinal area under investigation (Figure 1b). According to different resolutions (19, 37, 61 hexagons), the recording time of one complete recording ranged from 2 to 7 min involving averaging of several short m-sequence cycles (each 54 s long) to improve the signal-to-noise ratio. Responses originated from a scanned area 30° in diameter centred around the fovea. For a resolution of 19 elements in the cSLO mode, this diameter corresponds to the central 19 elements in monitor stimulation when a resolution of 61 elements is used. Stimulus presentation followed the principle of Maxwellian view. To reduce the amount of light scattering in the eye, modification of laser power became necessary. Since the HRA refers to monochromatic light, luminance cannot be specified by cd/m2 used to describe a wavelength spectrum, but only by power/area. Attenuation of the maximum laser power output from 84 to 0.4 μW was achieved by a Clarlet F 80 filter (ZEISS, Aalen, Germany) placed in front of the objective. Laser power was measured with a PD2-A monitor (Ophir Optronics, Jerusalem, Israel). Reducing the power of the infrared diode laser by only 4%, the original quality of the fundus image was maintained.
(a) Multifocal ERG set-up with confocal scanning laser ophthalmoscope for recording with simultaneous fundus monitoring. (b) Right fundus with visualized stimulus pattern (37 hexagons) at the posterior pole. (c) Numbers of rings used for analysis. The resolution of 19 hexagons comprises rings 1–3, that of 37 hexagons comprises rings 1–4, and analysis includes an additional ring 5 in the resolution of 61 hexagons.
Conventional stimulation
The stimulus array consisted of 61 hexagons (Figure 1c) and was presented on a Sony GDM colour monitor driven at a frame rate of 60 Hz. The RETIscan monitor mode involves four interspersed dark frames; consequently, the time period between each stimulus frame is 83 ms. The stimulus array covered the central 58° of the retina. A complete recording comprised eight 37-s cycles of short m-sequence stimulation averaged together to increase signal-to-noise ratio. Luminance was 119cd/m2 for white and 1 cd/m2 for black hexagons, resulting in a Michelson contrast of 99%.
Gold foil electrodes were used for signal derivation unless otherwise indicated. The pupil size was natural.6 The signal was bandpass filtered (10–100 Hz), amplified (× 50 000) and recorded at sampling intervals of either 1 ms (monitor mode) or 2 ms (cSLO mode). An artefact rejection technique was applied rerecording artefact-containing response traces immediately during the proceeding of the short m-sequence cycle.
The first-order kernel was analysed, and to evaluate the measurement of cell receptor density responses were averaged over concentric rings around the fovea.
Subjects
Three patients differing in type and topography of their retinal pathology and two normal subjects without any known eye diseases were examined. As to retinal diseases we chose patients with well-defined pathologies to clearly perceive restrictions of the cSLO mode. We did not include patients with fixation pathology since we were primarily interested in the performance of the cSLO in detecting localized dysfunction. This would have been more difficult if unstable fixation had obscured local response characteristics. Patient 1 (50-year-old male) diagnosed with retinitis pigmentosa showed predominantly an affection of the peripheral retina. Patient 2 (57-year-old male) suffering from pericentral retinitis pigmentosa revealed patchy pericentral chorioretinal atrophy. Retinal pathology in patient 3 (39-year-old female) with autosomal dominant optic atrophy was not visible. Procedures followed the tenets of the Declaration of Helsinki and informed consent was obtained from all subjects prior to examination.
Results
Normal mfERG topography
In consideration of viewing distance and dimensions of the stimulus elements, the central 19 hexagons forming rings 1–3 of the monitor stimulus array approximately correspond to the scanned area of the HRA. Note that the single stimulus patches cannot be compared exactly as the cSLO mode employed unscaled hexagons. The central element subtended 0.3° less than the monitor stimulus, ring 2 was 0.5° and ring 3 was 0.6° smaller in diameter.
In the resolution of 19 hexagons (Figure 2a) clearly shaped waveforms showing a distinct negative and positive deflection could be derived for each stimulated retinal area.7 Looking at response densities in nV divided by the area of the respective stimulated retinal location, amplitude values in the cSLO recording were lower than in the monitor mode (Figure 2c). HRA stimulation resulted in a decrease of amplitude of 28 nV/deg2 (Figure 2b) whereas conventional mfERG recording comprised 81 nV/deg2 (Figure 2d) calculated from rings 1–3. Latencies obtained with the HRA were prolonged compared to the monitor stimulation. The outer part of hexagon 12 which was scanned on the optic nerve head presented a minimum amplitude value.
Recording from the right eye of a normal subject with cSLO (a) and monitor (c) stimulation. The stimulus array of the cSLO covered the central 30° and the array of the monitor stimulation spanned the central 58°. Here, the central 19 elements (rings 1–3 as indicated by Figure 1c) extend to 30° and therefore correspond to the responses shown in the cSLO record. Responses are averaged over concentric rings in cSLO (b) and monitor mode (d) with numbers indicating rings 1–3 or 1–5, respectively. Response density values are shown in the left column and latency values of the first positive peak in the right column.
The responses derived from 61 retinal areas (Figure 3a) also showed lower values than in monitor recordings (Figure 3c, d). Amplitudes diminished by 31 nV/deg2 starting from a maximum value of 50.3 nV/deg2 (Figure 3b). In contrast to the array of 19 elements the blind spot was not detectable. Waveform shapes were highly variable across the stimulated field and partly lacked a distinct trough and peak.
Trace array of a normal right eye recorded with a resolution of 61 hexagons in cSLO (a) and monitor (c) mode. Note that the diameter of the cSLO stimulus array (30° visual angle) measures only half of the stimulus used in the monitor mode (58° visual angle). Responses are averaged over concentric rings. Ring numbers (1–5), response density values (left column), and latency values of the first positive peak (right column) are shown for cSLO (b) and conventional monitor (d) stimulation.
Retinitis pigmentosa (peripheral type)
Funduscopic examination of a 48-year-old male patient revealed peripheral bone spicule pigmentation, a waxy optic atrophy and mild attenuation of arteries (Figure 4a). Visual acuity was 20/20. Ganzfeld-ERG amplitudes were markedly reduced and Octopus perimetry exhibited a concentric visual field constriction (Figure 4b). In cSLO stimulation (Figure 4c) amplitude decrease is not as clear because of a relatively greater response contribution from central parts of the retina. The trace array of 61 focal ERGs obtained with the conventional mfERG (Figure 4d) underlines the visual field defect by displaying a loss of amplitude towards the periphery (note that a DTL-electrode was used for this recording).8,9 In the periphery, response reduction is easier to detect. It relates to the retinal dysfunction revealed by the perimetry findings. However, as the Octopus perimetry represents a threshold profile, a direct comparison to mfERG results that yield a suprathreshold response profile is not necessarily possible.10 In both mfERG methods, latencies of the first positive peak were prolonged.
(a) Fundus photograph (left eye) of a patient with retinitis pigmentosa showing focal hyperpigmentation, attenuated vessels and atrophy peripheral to the temporal vascular arcade. (b) Octopus perimetry results from a test area measuring 60° visual angle in diameter exhibit a concentric visual field constriction. (c) The cSLO recording (stimulus diameter 30° visual angle) reflects an amplitude decrease with increasing eccentricity. (d) With monitor mode stimulation the signal loss towards the periphery is more pronounced as more of the dysfunctional regions fall into the stimulus array that was approximately 58° visual angle in diameter.
Pericentral retinitis pigmentosa
Biomicroscopy of a 55-year-old patient showed pericentral atrophic and pigmented patches of the retinal pigment epithelium. Visual acuity was 20/20 and visual field testing revealed a pericentral scotoma (Figure 5a). Ganzfeld-ERG showed a reduced rod response. As the perimetry findings suggest the foveal response was considered to be small in this patient. Thus, a resolution of 37 hexagons was chosen in order to elicit a distinguishable central response (Figure 5b). This implies that response density values cannot be compared elementwise as in the higher resolution of 61 hexagons since the central 19 hexagons now exceed the scanned area in cSLO recording. Higher amplitudes in the periphery as well as signal loss in the pericentral retina were detected by the conventional method, the area of dysfunction approximately corresponding to the pericentral scotoma presented by Octopus perimetry. Again, in perimetry a detection threshold is examined while in mfERG suprathreshold responses are calculated. In addition, responses recorded as a result of the illumination of a defined fundus area may not only stem from that area alone but may present stray light responses of the surroundings. This applies in particular to the fundus areas with increased reflectance (ie areas of chorioretinal atrophy). A marked peripheral increase in the fundus-monitored ERG amplitudes was hard to detect showing only few responses in the outermost ring (Figure 5c) because an area subtending only 15° centre-to-periphery was stimulated. Presumably, stray light induced activity prevailed from the dark surround of the stimulus and adaptation field contaminating the response waveforms.
(a) A patient with pericentral retinal regeneration shows a pericentral scotoma in Octopus perimetry (test area diameter subtends 60° visual angle) in his left eye. (b) Trace array obtained with conventional mfERG recording. With 58° visual angle, the diameter of the array corresponds approximately to the Octopus perimetry. Central retinal responses are severely reduced. (c) In cSLO recording it is only at some locations within the outermost ring that responses could be detected as nearly all of the stimulus elements (the diameter of the stimulus array measured 30° visual angle) fall into the dysfunctional area.
Dominant optic atrophy
A 35-year-old patient with autosomal dominant bilateral temporal optic atrophy and a visual acuity of 20/40 underwent mfERG examination. Colour arrangement tests and an enlarged matching range with Engelking's blue–green equation documented tritan confusion. Visual field testing was unremarkable. The trace arrays of 19 and 61 focal ERGs obtained with the conventional (Figure 6a) and fundus-monitored (Figure 6b) mfERG, respectively, demonstrate a normal response distribution. Averages of response waveforms represented a normal functional topography showing highest amplitudes in the foveal area decreasing towards the peripheral retina as expected from a first-order kernel analysis in optic nerve disease. Judging on the condition of the outer retinal layers, reference values of the patient's healthy eye are required since even in the first-order kernel response there may be some amplitude changes11 best revealed in interocular comparison.12 However, to evaluate optic nerve function, a second-order kernel analysis would be more promising.
Both monitor (a) and fundus-controlled (b) stimulation show maximum central responses and peripherally decreasing amplitudes in a patient with dominant optic atrophy. Records were obtained from the patient's right eye. The central 19 traces of the monitor mode correspond to the traces in (b). Stimulus diameters measured 58° (a) and 30° (b) visual angle.
Discussion
MfERGs with simultaneous fundus visualization were recorded using a cSLO that generates the stimulus with an argon-green laser beam and images the retinal position of the stimulus by means of an infrared diode laser beam. Results were qualitatively compared to the standard monitor stimulation.
A previous study showed that fundus-monitored recording is possible by using an infrared television fundus camera.13 Employment of a cSLO allowed for fundus-monitored recordings of visual evoked potentials14 and pattern electroretinograms15 and has also been used for recording of mfERGs in animals.16 A recent study on the reproducibility of the cSLO in a normal population showed a high degree of variability within and between subjects considered to be a substantial limitation of this method.17
Clinical application of mfERG recording with simultaneous fundus monitoring from humans has not been evaluated so far, although it might contribute valuable information about recording quality as the exact position of the stimulus on the retina can be determined.
For exact focusing of the image in the cSLO stimulation mode no correction for refractive errors is required. In contrast, the RETIscan system does not provide the possibility of directly focusing the image on the retina in monitor mode so that refracting the subjects for best visual acuity is necessary. Throughout the entire duration of the examination, the exact localization of the stimulus on the fundus was monitored with the cSLO system. Whenever a patient's fixation deviated from the fixation mark, verbal encouragement to refixate or adjustment to the correct retinal location assured the most constant positioning possible. When the fovea moved out of the central element, the m-sequence run was stopped and rerecorded.
In contrast to monitor stimulation cSLO-derived waveforms in high resolution and from patients with fixation problems, especially patient 2, were highly variable. The stimulus image is focused on the retina in Maxwellian view after the argon-green laser beam passed a 3 mm illumination aperture in the HRA optics. By making small head movements on the headrest, light may partially aim at the inner edges of the patient's iris causing different retinal activity on different locations of the same eccentricity in the stimulus array. Thus, recording from patients in miosis with a view to patients' comfort and also a shorter examination procedure should be considered carefully.
Variability between waveforms lying on the same eccentricity may partly account for the disparity in latencies between the two stimulation modes. Calculating ring averages from waveforms with different shapes becomes imprecise and therefore renders the latency calculation somewhat inaccurate.17
In patients as well as in normal subjects response density values obtained by the cSLO mode were generally lower than with the monitor mode. Working on a frame rate of 10 Hz, the stimulus build-up speed achieved by laser stimulation is very slow. To integrate retinal activity over a reasonable time period, a raise of the frame rate resulting in faster scanning of the hexagonal elements would be helpful. Furthermore, lower amplitude values result in a lower overall amplitude range calculated from the centre to the periphery of the scanned area rendering it harder to detect abnormal amplitude values in disease. Another shortcoming of the system in its current state is that only 37 retinal locations could be tested with an acceptable signal-to-noise ratio that may be too low to detect regional retinal dysfunction.
The findings show that recording mfERGs under fundus monitoring in a clinical surrounding is possible. An evaluation of the cSLO stimulation mode on a larger patient population is necessary in order to substantiate our preliminary results. Being aware of the poor reproducibility in normal subjects17 and the current technical limitations, the system demands further technical development before routine clinical application becomes feasible.
The authors have no proprietary interests in any of the products mentioned.
References
Sutter EE, Tran D . The field topography of ERG components in man—I. The photopic luminance response. Vis Res 1992; 32: 433–436.
Sutter EE . The fast m-transform: a fast computation of cross-correlations with binary m-sequences. SIAM J Comput 1991; 20: 686–694.
Sutter EE . A deterministic approach to nonlinear systems analysis. In: Pinter RB, Nabet B (eds). Nonlinear Vision. CRC Press: Cleveland, 1992, pp 171–220.
Kutschbach E . Method for the Multifocal ERG using Short Length and Corrected M-sequences. Roland Consult, Elektrophysiologische Diagnostik Systeme: Wiesbaden, 1997, pp 1–11.
Holz FG, Bellmann C, Rohrschneider K, Burk RO, Völcker HE . Simultaneous confocal scanning laser fluorescein and indocyanine green angiography. Am J Ophthalmol 1998; 125: 227–236.
Park S, Keating D, Williamson TH, Jay JL, Elliott AT . Functional imaging of the retina using the multifocal electroretinograph: a control study. Br J Ophthalmol 1998; 80: 831–834.
Hood DC, Seiple W, Holopigian K, Greenstein V . A comparison of the components of the multifocal and full-field ERGs. Vis Neurosci 1997; 14: 533–544.
Chan HL, Brown B . Investigation of retinitis pigmentosa using the multifocal electroretinogram. Ophthal Physiol Opt 1998; 18: 335–350.
Seeliger M, Kretschmann U, Apfelstedt-Sylla E, Ruther K, Zrenner E . Multifocal electroretinography in retinitis pigmentosa. Am J Ophthalmol 1998; 125: 214–226.
Hood DC, Holopigian K, Greenstein V, Seiple W, Li J, Sutter EE et al. Assessment of local retinal function in patients with retinitis pigmentosa using the multi-focal ERG technique. Vis. Res 1997; 38: 163–179.
Bearse MA, Sim D, Sutter EE, Stamper R, Leiberman M . Application of the multifocal ERG to glaucoma. Invest Ophthalmol Vis Sci 1996; 37: 511.
Hasegawa S, Takagi M, Usui T, Takada R, Abe H . Waveform changes of the first-order multifocal electroretinogram in patients with glaucoma. Invest Ophthalmol Vis Sci 2000; 41: 1597–1603.
Kondo M, Miyake Y, Horiguchi M, Suzuki S, Tanikawa A . Recording multifocal electroretinograms with fundus monitoring. Invest Ophthalmol Vis Sci 1997; 38: 1049–1052.
Katsumi O, Timberlake GT, Hirose T, Van de Velde FJ, Sakane H . Recording pattern reversal visual evoked response with the scanning laser ophthalmoscope. Acta Ophthalmol 1989; 67: 243–248.
Horn F, Korth M . Differences between pattern-evoked electroretinograms obtained by a scanning laser ophthalmoscope and by a mechanical mirror system. Doc Ophthalmol 1994; 88: 65–75.
Seeliger MW, Narfström K . Functional assessment of the regional distribution of disease in a cat model of hereditary retinal degeneration. Invest Ophthalmol Vis Sci 2000; 41: 1998–2005.
Poloschek CM, Friede T, Krastel H, Holz FG . Multifokales Elektroretinogramm mittels konfokalem Scanning Laser Ophthalmoskop: Vergleich mit Monitorstimulation. Ophthalmologe 2002; 99: 457–463.
Author information
Authors and Affiliations
Corresponding author
Rights and permissions
About this article
Cite this article
Poloschek, C., Rupp, V., Krastel, H. et al. Multifocal ERG recording with simultaneous fundus monitoring using a confocal scanning laser ophthalmoscope. Eye 17, 159–166 (2003). https://doi.org/10.1038/sj.eye.6700294
Published:
Issue Date:
DOI: https://doi.org/10.1038/sj.eye.6700294
Keywords
This article is cited by
-
Effects of unsteady fixation on multifocal electroretinogram (mfERG)
Graefe's Archive for Clinical and Experimental Ophthalmology (2006)
-
Age-related Macular Disease: How to assess the retina using scanning laser techniques?
Aging Clinical and Experimental Research (2005)