Key Points
-
This article aims to guide readers in taking the correct purchase/use decision of digital radiographic equipment, based on scientific evidence, by achieving a proper balance between diagnostic quality and radiation dose.
-
There is a lower radiation dose to the salivary gland when indirect digital rather than direct cephalometric radiography is carried out.
-
Their image quality is comparable for both direct and indirect digital cephalometric radiography.
Abstract
Aim The aim of this study was to measure organ doses and calculate the effective dose for indirect and direct digital cephalometric exposures.
Material and methods Indirect digital cephalometric exposures were made of a Rando® phantom head using a Cranex Tome® multipurpose unit with storage phosphor plates from Agfa and the direct digital (Charge Coupled Device, CCD) exposures were made with a Proline Ceph CM® unit. Exposure settings were 70 kV and 4 mAs for indirect digital exposures. Direct digital exposures were made with 70 kV, 10 mA and a total scanning time of 23 s. TLD700® dosemeters were used to measure organ doses, and the effective doses were calculated with (effective dosesal) and without inclusion of the salivary glands. A pilot study was carried out to compare diagnostic image quality of both imaging modalities.
Results Effective doses were 1.7 μSv for direct digital and 1.6 μSv for indirect digital cephalometric imaging. When salivary glands were included in the calculation, effective dosessal were 3.4 μSv and 2.2 μSv respectively. Organ doses were higher for direct digital imaging, except for the thyroid gland, where the organ doses were comparable. Diagnostic image quality of indirect and direct digital cephalometric images seemed comparable.
Conclusion Effective dose and effective dosesal were higher for direct digital cephalometric exposure compared with indirect digital exposure. Organ doses were higher for direct digital cephalography. From preliminary data, it may be presumed that diagnostic image quality of indirect and direct digital cephalometric images are comparable.
Similar content being viewed by others
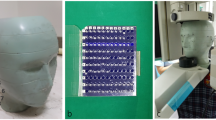
Introduction
Digital equipment for radiographic exposures was introduced to dentistry in the late 1980s (RadioVisioGraphy®, Trophy, Vincennes, France). The first systems were based on the charge-coupled device technology (CCD), which can also be found in camera systems. With this technique, radiation is detected by the sensor and converted into digital data, which are sent immediately to a computer. The radiographic image is almost 'real time' shown on a computer monitor and is therefore also called 'direct digital radiography'. In the early 1990s, digital storage phosphor systems took over from medical radiology. Storage phosphor plates (SPP) capture radiation energy for a certain period and can be read out by a laser scanner, which converts radiation energy into light energy that is measured and translated into digital data. Because of the delay caused by the scanning procedure, the digital storage phosphor technique is also called 'indirect digital radiography'.
A technical limitation of extra-oral direct digital radiography is the very small active area of the CCD sensor. This is a minor problem for intra-oral digital radiography, where the active area of the sensor remains somewhat smaller than a conventional intra-oral film. For static extraoral radiography, such as anteroposterior and cephalometric projections however, a linear scanning procedure is usually applied to cover the whole area. This lengthens the exposure time considerably.
Among other advantages, such as the possibility to digitally manipulate the radiographic data, digital radiography can possibly reduce radiation dose compared with conventional radiography. Digital sensors (both direct and indirect) should be more sensitive for radiation energy, which allows a lowering of radiation dose. This is especially true for intra-oral radiography, where a significant reduction of radiation dose has been reported.1,2,3,4,5 For extraoral digital radiography however, a smaller reduction can be expected because of the already low dose thanks to the use of intensifying screens and fast film-screen combinations in conventional radiography. For digital panoramic radiography, different amounts of dose reduction have been reported.6,7,8 In a previous report9, comparing conventional and indirect digital cephalometric radiography, it was found that image quality of digital and conventional radiographs was not significantly different. However, image quality of indirect digital radiographs was more stable for variations in exposure parameters than conventional radiographs. Because of the different exposure technique (linear scanning procedure) used in direct digital cephalometric radiography, a direct comparison or interpolation of radiation doses is impossible. The current study, therefore, was designed to measure organ doses for both direct and indirect digital exposure techniques for cephalometric exposures. A pilot study was also performed to compare the diagnostic image quality of the two digital imaging techniques.
Material and methods
Exposures were made with storage phosphor plates (24 cm × 30 cm, ADCC MD® plate Agfa, Mortsel, Belgium) using the cephalometric programme of the Cranex Tome® multipurpose radiation unit (Soredex, Helsinki, Finland). Exposure settings were 70 kV and 4 mAs, considered as being the settings used for an average person in daily practice. A custom-made lead collimator was placed in front of the radiation tube to limit the exposure area (Fig. 1). The lead collimator was constructed from a 3 mm thick piece of lead and attached to the radiation unit in front of the radiation source. In a previous study,10 it was found that this collimator could reduce the field size by 55% and the effective radiation dose by almost 50%. The direct digital exposures were made with the Proline® Ceph CM unit (Planmeca, Helsinki, Finland) which has a cephalometric exposure area of 18 cm × 24 cm. The same lead collimator was applied, taking care to collimate down to the same region of the skull with both cephalometric units. Exposure settings were 70 kV and 10 mA. The total exposure time for the linear scanning procedure was 23 s. These are also the settings used for an average person in daily practice.
A Rando® phantom head (Alderson Research Laboratories, NY, USA) (Fig. 2) representing an average man was exposed. The Rando® phantom head consisted of a human skull and vertebrae surrounded and filled with tissue-equivalent material. It is a generally accepted method to measure absorbed radiation doses in different organs of the human body.11,12,13,14,15,16,17 The skull is cut into horizontal slices and every slice contains holes that can be filled with dosemeters. In the present study, dosemeters were put in the submandibular glands, parotid glands, bone marrow of the ascending ramus, pituitary gland, corpus callosum, frontal brain lobe, cerebellum, thyroid gland, lenses of the eyes and skin. Two dosemeters were used for each site and each exposure was repeated ten times. The dosemeters were of the LiF:Mg,Ti TLD-700® type (Bicron NE, Solon, USA) (containing only Li-7, without Li-6). They consisted of ribbons of 3 × 3 × 0.15 mm3 and have a standard deviation of 10% (variability of the resulting doses after manipulation and reading out procedures). They were read out with a fully-automated Harshaw 6600® reader (Bicron NE, Solon, USA) after correction for atmospheric pressure, temperature and air humidity. The resulting data were divided by 10 and averaged for the two dosemeters per site. A number of dosemeters (five per session) underwent the same procedure, except for the irradiation, to record the background radiation (around 30 to 40 μGy). These values were subtracted from the recorded data.
The data of the radiation doses absorbed by different organs were then used for calculation of the effective radiation dose, which is an indication of the impact of a certain radiation exposure on the whole human body. The formula takes the variation in sensitivity towards radiation of the different organs into account. Whereas absorbed radiation doses are expressed in (μ)Gy, effective dose is expressed in (μ)Sv. The effective dose was calculated using the formula: 'Deff = ΣDabs.WF' with Deff being the effective dose, Dabs the absorbed organ doses and WF the weighting factors as determined by ICRP60.18 The effective dose was calculated both with ('effective dosesal') and without ('effective dose') inclusion of the salivary glands as part of the remainder organs.19 Following the ICRP regulations, salivary glands are not considered as organs sensitive for the detrimental effects of radiation. A number of studies,20,21,22,23 however, indicate a possible relationship between ionising radiation and salivary gland cancers. A weighting factor of 0.05 was assigned to the average of the remainder organs (pituitary gland, cerebellum, frontal brain lobe, corpus callosum and salivary glands).
Because skin dose and bone marrow dose were only measured locally, a formula described by Huda and Sandison24 was used to calculate the whole body skin and bone marrow doses. The bone marrow dose was also used for the bone surface dose. For those organs not measured in the present study, the absorbed dose was assumed to be zero.
In order to compare the diagnostic image quality of the indirect and direct digital cephalometric radiographs, a pilot study was performed by four observers. The visibility of six different cephalometric landmarks (nasion, orbitale, A point, B point, pogonion and gonion) was scored on a 5-point scale (1 = minimal visibility, 5 = maximal visibility) for the two digital techniques. In both cases, the radiographs taken from the phantom head were shown on a computer monitor (17-inch screen, 24-bit colour depth, resolution of 1024 × 768 pixels, dimmed ambient light). The observers were blinded for the type of panoramic radiographs (direct or indirect digital) and the radiographs were shown in random order. The ratings were repeated with a 14-day interval. The data were statistically analysed using the Kruskal-Wallis ANOVA (Statistica®, Tulsa, OK, USA) with observer and panoramic unit as independent variables and cephalometric landmark and time of assessment as dependent variables.
Results
The results of the dose measurements and the resulting effective dose are shown in Table 1. In the table, the results for ten repeated exposures are divided by 10 after subtraction of the background radiation (30 to 40 μGy). The effective doses are 1.6 μSv for indirect digital cephalometric exposure and 1.7 for direct digital cephalometric exposure. The corresponding effective dosessal are 2.2 μSv and 3.4 μSv respectively.
The diagnostic image quality of the two digital techniques were comparable for the abovementioned cephalometric landmarks, with good visibility (average score > 3) for nasion, orbitale, B-point, pogonion and gonion, and poorer visibility (average score of 2.81) for A-point. Inter- and intra-observer differences were also not statistically significant.
Discussion
The inclusion of the salivary gland tissue in the calculation of the effective dose has a remarkable effect. Whereas the effective doses of indirect and direct exposures are comparable (1.6 μSv vs 1.7 μSv respectively, which is a difference of 9%) when the salivary tissue is not included, the difference is larger when the salivary tissue is included (2.2 μSv compared with 3.4 μSv, or 56%).
When absorbed organ doses were considered, an overall higher organ dose was found for direct digital cephalometry, except for the dose to the thyroid gland, which was comparable. For other organs, absorbed doses of up to 19 times higher (eye lens tube side) could be found for direct digital imaging. This has, however, not a large effect on the effective dose because the doses to the lenses of the eyes are not included in the calculation (deterministic effect, with threshold dose of 2 Gy25). Furthermore, the skin and bone marrow dose contribute only minimally to the effective dose due to the use of the correction factors.24 Thus, the organs most involved in the calculation of the effective dose are the thyroid gland, the brain tissue and, for the effective dosesal, the salivary glands. This explains also the large effect of the inclusion of the salivary gland tissue on the effective dose.
The difference in absorbed organ doses between indirect and direct digital cephalometric exposures can probably be explained by the different nature of the exposure technique. For the direct digital imaging technique, a linear scanning procedure is used, whereas a short exposure 'shot' is used for the indirect digital technique. The longer time required by the scanning procedure also implies a risk of movement artefacts, especially for children.
As a more general remark, it should be mentioned that differences in radiation dose could be due to individual anatomical variations. However, as the same phantom set-up was used for both digital imaging techniques, at least a relative comparison between both methods can be made.
Furthermore, a custom-made lead collimator was used for cephalometric exposures, which means that higher doses will be achieved in clinical practice where this collimator is not used.
A study by Visser et al. in 200126 on dosimetric measurements for direct digital and conventional cephalometric radiography, yielded an effective dose for direct digital cephalometric exposure of 1.1 μSv, which is comparable to our result. A Siemens Orthophos DS Ceph® unit (Sirona Dental, Bietigheim-Bissingen) was used. The exposure settings were 73 kV, 15 mA and 15.8 s. Additional collimation was not used.
A preliminary evaluation of the diagnostic image quality of both digital imaging systems was performed in a pilot study by assessing the visibility of six cephalometric landmarks (nasion, orbitale, A-point, B-point, pogonion, gonion). These landmarks were selected because they are relatively independent of the possible superimposition of anatomical structures.27 For both imaging techniques, relatively high scores were assigned to nasion, orbitale, B-point, pogonion and gonion. The A-point was scored lower for both techniques. Previous studies already showed that diagnostic image quality of cephalometric radiographs is not so much dependent on physical properties but rather on observer performance and pattern recognition.28,29 A study by Liu et al.30 showed that collimation of cephalometric radiographs leads to improved contrast and diagnostic image quality. It should also be kept in mind that a phantom head was used to evaluate the image quality, which is not subject to possible movement artefacts due to the almost 60 times longer exposure time for direct digital exposures.
Conclusion
In conclusion, higher organ doses are found for direct digital cephalometric radiography, except for the thyroid gland. Depending on the inclusion of the salivary gland tissue in the calculation of the effective dose, effective doses for direct digital cephalometric imaging are 9% (1.7 μSv compared with 1.6 μSv) or 56% higher (effective dosesal 3.4 μSv compared with 2.2 μSv) than for indirect digital cephalometric exposures. Furthermore, from preliminary data, it seems that diagnostic image quality of both digital imaging techniques is comparable.
References
Brettle DS, Workman A, Ellwood RP, Launders JH, Horner K, Davies RM . The imaging performance of a storage phosphor system for dental radiography. Br J Radiol 1996; 69: 256–261.
Lim KF, Loh EE-M, Hong YH . Intra-oral computed radiography — an in vitro evaluation. J Dent 1996; 24: 359–364.
Velders XL, Sanderink GCH, van der Stelt PF . Dose reduction of two digital sensor systems measuring file lengths. Oral Surg Oral Med Oral Pathol Oral Radiol Endod 1996; 81: 607–612.
Huysmans MCD, Hintze H, Wenzel A . Effect of exposure time on in vitro caries diagnosis using the Digora system. Eur J Oral Sci 1997; 105: 15–20.
Yoshiura K, Kawazu T, Chikui T, Tatsumi M, Tokumori K, Tanaka T, Kanda S . Assessment of image quality in dental radiography, part 2: optimum exposure conditions for detection of small mass changes in 6 intra-oral radiography systems. Oral Surg Oral Med Oral Pathol Oral Radiol Endod 1999; 87: 123–129.
Dula K, Sanderink G, van der Stelt PF, Mini R, Buser D . Effects of dose reduction on the detectability of standardized radiolucent lesions in digital panoramic radiography. Oral Surg Oral Med Oral Pathol Oral Radiol Endod 1998; 86: 227–233.
Farman TT, Farman AG, Kelly MS, Firriolo FJ, Yancey JM, Stewart AV . Charge-coupled device panoramic radiography: effect of beam energy on radiation exposure. Dentomaxillofac Radiol 1998; 27: 36–40.
Dannewitz B, Hassfeld S, Eickholz P, Muhling J . Effect of dose reduction in digital dental panoramic radiography on image quality. Dentomaxillofac Radiol 2002; 31: 50–55.
Gijbels F, Bou Serhal C, Willems G, Bosmans H, Sanderink G, Persoons M, Jacobs R . Diagnostic yield of conventional and digital cephalometric images: a human cadaver study. Dentomaxillofac Radiol 2001; 30: 101–105.
Gijbels F, Sanderink G, Wyatt J, Van Dam J, Nowak B, Jacobs R . Radiation doses of collimated vs non-collimated cephalometric exposures. Dentomaxillofac Radiol 2003; 32: 128–133.
Wall BF, Fisher ES, Paynter R, Hudson A, Bird PD . Doses to patients from pantomographic and conventional dental radiography. Br J Radiol 1979; 52: 727–734.
Velders XL, van Aken J, van der Stelt PF . Absorbed dose to organs in the head and neck from bitewing radiography. Dentomaxillofac Radiol 1991; 20: 161–165.
Velders XL, van Aken J, van der Stelt PF . Risk assessment from bitewing radiography. Dentomaxillofac Radiol 1991; 20: 209–213.
Gilda JE, Maillie HD . Dosimetry of absorbed radiation in radiographic cephalometry. Oral Surg Oral Med Oral Pathol 1992; 73: 638–643.
Hayakawa Y, Fujimori H, Kuroyanagi K . Absorbed doses with intra-oral radiography. Function of various technical parameters. Oral Surg Oral Med Oral Pathol 1993; 76: 519–524.
Farman TT, Farman AG, Kelly MS, Firriolo FJ, Yancey JM, Stewart AV . Charge-coupled device panoramic radiography; effect of beam energy on radiation exposure. Dentomaxillofac Radiol 1998; 27: 36–40.
Bou Serhal C, Jacobs R, Gijbels F, Bosmans H, Hermans R, Quirynen M, van Steenberghe D . Absorbed doses from spiral CT and conventional spiral tomography: a phantom vs. cadaver study. Clin Oral Implants Res 2001; 12: 473–478.
International Commission on Radiological Protection. Recommendations of the International Commission on Radiological Protection. ICRP Publication No. 60. Ann. ICRP 1991; 21: 1–3.
Lecomber AR, Downes SL, Mokhtari M, Faulkner K . Optimisation of patient doses in programmable dental panoramic radiography. Dentomaxillofac Radiol 2000; 29: 107–112.
Preston-Martin S, Henderson BE, Bernstein L . Medical and dental x rays as risk factors for recently diagnosed tumors of the head. Natl Cancer Inst Monogr 1985; 69: 175–179.
Preston-Martin S, Thomas DC, White SC, Cohen D . Prior exposure to medical and dental x-rays related to tumors of the parotid gland. J Natl Cancer Inst 1988; 80: 943–949.
Preston-Martin S, White SC . Brain and salivary gland tumors related to prior dental radiography: implications for current practice. J Am Dent Assoc 1990; 120: 151–158.
Horn-Ross PL, Ljung BM, Morrow M . Environmental factors and the risk of salivary gland cancer. Epidemiol 1997; 8: 414–419.
Huda W, Sandison G . Estimation of mean organ doses in diagnostic radiology from Rando phantom measurements. Health Physics 1984; 47: 463–467.
ICRP Publication 41. Nonstochastic effects of ionizing radiation. Ann ICRP 1984; 14: paragraph 3.
Visser H, Rödig T, Hermann K-P . Dose reduction by direct-digital cephalometric radiography. Angle Orthod 2001; 71: 159–163.
Trpkova B, Major P, Prasad N, Nebbe B . Cephalometric landmarks identification and reproducibility: A Meta Analysis. Am J Orthod Dentofac Orthoped 1997; 112: 165–170.
Näslund E-B, Kruger M, Petersson A, Hansen K . Analysis of low-dose digital lateral cephalometric radiographs. Dentomaxillofac Radiol 1998; 27: 136–139.
Rossman K, Wiley B . The central problem in the study of radiographic image quality. Radiol 1970; 96: 113–118.
Liu YT, Gravely JF . The effect of beam collimation in lateral radiographic cephalometry. Br J Orthod 1991; 18: 119–124.
Acknowledgements
Reinhilde Jacobs is a postdoctoral researcher of the Fund for Scientific Research Flanders.
Author information
Authors and Affiliations
Corresponding author
Additional information
Refereed Paper
Rights and permissions
About this article
Cite this article
Gijbels, F., Sanderink, G., Wyatt, J. et al. Radiation doses of indirect and direct digital cephalometric radiography. Br Dent J 197, 149–152 (2004). https://doi.org/10.1038/sj.bdj.4811532
Received:
Accepted:
Published:
Issue Date:
DOI: https://doi.org/10.1038/sj.bdj.4811532
This article is cited by
-
Modern 3D cephalometry in pediatric orthodontics—downsizing the FOV and development of a new 3D cephalometric analysis within a minimized large FOV for dose reduction
Clinical Oral Investigations (2021)
-
Pediatric cleft palate patients show a 3- to 5-fold increase in cumulative radiation exposure from dental radiology compared with an age- and gender-matched population: a retrospective cohort study
Clinical Oral Investigations (2018)
-
Cone beam computed tomography
international journal of stomatology & occlusion medicine (2015)
-
Quantitative Evaluation of Patient Movement during Simulated Acquisition of Cephalometric Radiographs
Journal of Digital Imaging (2011)
-
Modern dental imaging: a review of the current technology and clinical applications in dental practice
European Radiology (2010)