Phaseolus vulgaris alphaendornavirus-1 is frequent in bean germplasm in Slovakia and shows low molecular variability
- 1Faculty of Natural Sciences, University of Ss. Cyril and Methodius, Trnava, Slovakia
- 2National Agricultural and Food Centre, Research Institute of Plant Production, Piešťany, Slovakia
- 3Institute of Virology, Biomedical Research Center of the Slovak Academy of Sciences, Bratislava, Slovakia
- 4Department of Microbiology and Virology, Comenius University in Bratislava, Bratislava, Slovakia
- 5Université de Bordeaux, Institut National de recherche pour l’agriculture, l’alimentation et l’environnement (INRAE), UMR 1332 Biologie du Fruit et Pathologie, Villenave d'Ornon, France
Phaseolus vulgaris alphaendornavirus-1 (PvEV-1, family Endornaviridae) was identified by ribodepleted total RNA high-throughput sequencing in the virome of two bean plants (Phaseouls vulgaris L.) grown in a garden in western Slovakia. Two nearly complete PvEV-1 genomes (ca. 14.06 kb, named PV1 and PV2) were assembled, showing 99.9% nucleotide identity, while their nucleotide identity with the reference PvEV-1 genome (NC_039217) reached 98.4%. Two primer pairs spanning the viral helicase encoding region and sequence upstream of the RNA-dependent RNA polymerase were designed and used to confirm the presence of the virus in the original bean samples by RT-PCR. A subsequent search for PvEV-1 presence in Slovakia was focused on two groups of samples: 1) bean plants grown under open field conditions and sampled during the vegetation period and 2) bean accessions grown from seeds obtained from a Slovak and French bean germplasm collection. Based on RT-PCR results, 4 out of 15 bean samples from open fields and 12 out of 21 bean accessions from the curated germplasm collection tested PvEV-1-positive. Interestingly, sequencing of RT-PCR products revealed that all amplified isolates are identical in the two amplified genomic portion which is also identical to those of the PV1 and PV2 isolates. These results suggest a relatively high incidence of PvEV-1 in bean in Slovakia. This is the first evidence and characterization of PvEV-1 from bean plants in Europe.
Introduction
Plant viruses are generally considered to be pathogens, i.e., agents whose infection damages the fitness, yield or quality of their hosts (Malmstrom et al., 2011). In recent years, however, reports have been accumulating of infections involving plant viruses with no apparent detrimental effects on their hosts, referred to as latent, cryptic or persistent viruses (Roossinck, 2010). There has also been a suggestion that some viruses might even be beneficial to their plant hosts (Roossinck, 2015). Asymptomatic infections can result from tolerance, in which plants do not suffer from wild-type (high-titer) levels of virus replication, or from viral persistence, in which virus titers are reduced to avoid cytopathic effects and host damage (Takahashi et al., 2019).
The commonly occurring persistent viruses in plants include viruses from the Endornaviridae family. These viruses have been suggested to have very long evolutionary relationships with their plant hosts and are characterized by efficient seed and pollen (vertical) transmission (Okada et al., 2013; Candresse et al., 2016; Nordenstedt et al., 2017). With the single possible exception of the endornavirus associated with the 447 cytoplasmic male sterility in Vicia faba (broadbean) (Lefebvre et al., 1990; Pfeiffer et al., 1993; Pfeiffer, 1998), endornaviruses are not reported to cause any obvious symptoms or pathogenic effects. On the contrary, some observations suggest a beneficial effect of endornavirus infection on the host phenotype (Roossinck, 2010). It should however been noted that some fungi-infecting endornaviruses have tentatively been associated with hypovirulence phenotypes (Osaki et al., 2006; Yang et al., 2018; Zheng et al., 2019; Wang et al., 2020; Luo et al., 2022).
The Endornaviridae genome consists of a linear, single-stranded RNA molecule ranging from 9.8–17.6 kb in size depending on the particular endornavirus species (Roossinck et al., 2011; Valverde et al., 2019). Because of the lack of a coat protein gene, the RNA molecules are not encapsidated and the double-stranded replicative intermediates are commonly found in cytoplasmic membraneous vesicles in infected plant cells (Fukuhara, 2019).
Taxonomically, the Endornaviridae family comprises two genera, i.e., Alphaendornavirus and Betaendornavirus. While alphaendornaviruses are associated with the infection of plants, fungi and oomycetes, betaendornaviruses are to date only known from ascomycete fungi (Valverde et al., 2019).
Genomes of alphaendornaviruses contain a single open reading frame encoding a large polyprotein that possess several functional domains. While the RNA-dependent RNA polymerase (RdRp) domain is conserved and common among all known endornaviruses, other functional domains, such as helicase, methyltransferase, or glycosyltransferase, are found in some, but not all alphaendornavirus species (Li et al., 2021). To date, the exact function of these particular domains remains unclear. Some plant endornaviruses contain a site-specific break (nick) in the 5′-terminal part of ORF in the coding strand of the double-stranded replication intermediate (Roossinck et al., 2011; Sabanadzovic et al., 2016).
Vector-mediated or mechanical transmission of endornaviruses was not confirmed, and these viruses seem incapable of cell-to-cell movement and lack a movement protein. On the contrary the efficient vertical transmission of endornaviruses occurs at a high rate through seeds, pollen or fungal spores (Fukuhara, 2019).
The dsRNAs replicative intermediates from bean cv. Black Turtle (Wakarchuk and Hamilton, 1990) were later identified as Phaseolus vulgaris alphaendornavirus 1 (PvEV-1, Okada et al., 2013). The virus was subsequently detected in many other bean cultivars grown in several non-European locations (Khankhum et al., 2015; Nordenstedt et al., 2017).
Besides PvEV1, the genus Alphaendornavirus contains two other distantly related endornaviruses infecting beans, i.e., PvEV2 and PvEV3 (Okada et al., 2013; Okada et al., 2018). They exhibit ca 46%–47% nucleotide identity with PvEV1 and show some differences in the presence or organisation of polyprotein domains.
In this work, two nearly complete genomes of PvEV-1 were obtained from bean plants grown in Slovakia and analyzed by HTS. PvEV-1 infection was further confirmed both in additional field-grown bean plants, and in accessions obtained from a bean germplasm collection.
Material and methods
High-throughput sequencing (HTS)
Two bean plants (Phaseolus vulgaris, labelled as PV1 and PV2, both cv. Maxidor) growing in a private garden in Pezinok (western Slovakia, GPS coordinates: 48°18′11.6″N 17°16′35.6″E) were sampled in July 2021 and subjected to HTS.
Briefly, total RNAs were extracted from leaves using the Spectrum Plant Total RNA Kit (Sigma Aldrich, St. Louis, MO, United States). Ribosomal RNA was removed using the Zymo-Seq RiboFree Universal cDNA Kit (Zymo Research, Irvine, CA, United States). The ribosomal RNA-depleted total RNA samples were used for double-stranded cDNA synthesis using the SuperScript II kit (Thermo Fisher Scientific, Waltham, MA, United States). The cDNA was then purified with the 2.2 x AMPure XP beads and quantified with a Qubit 2.0 Fluorometer (Thermo Fisher Scientific, Waltham, MA, United States). Subsequently, the samples were processed with the transposon-based chemistry library preparation kit (Nextera XT, Illumina, San Diego, CA, United States). Low-cycle PCR and mutual indexing of the fragments was carried out. Fragments were purified with 1.8 x AMPure XP beads (Beckman Coulter, Brea, CA, United States) without size selection. The fragment size structure of the DNA library was assessed using the Agilent 2100 Bioanalyzer (Agilent Technologies, Santa Clara, CA, United States). An equimolar pool of 4 nM DNA libraries was denatured, diluted to 10 pM and sequenced (2 × 150 bp paired reads paired-end sequencing) on the Illumina MiSeq platform (Illumina, San Diego, CA, United States). High-quality trimmed reads were used for de novo assembly and contigs aligned to the viral genomes database (ftp://ftp.ncbi.nih.gov/genomes/Viruses/all.fna.tar.gz) using CLC Genomics Workbench 9.5.2 and Geneious v.8.1.9 softwares. Alternatively, the reads were mapped against selected full-length reference genome sequences of viruses identified in the previous step. Sanger sequencing of PCR products obtained by using PvEV-1-specific primers described below was used to validate two portions of the genome.
The nearly complete nucleotide sequences reported in this paper (missing 10 and 8, and 2 and 5 nucleotides, respectively, at the 5′ and 3′extremities, for PV1 and PV2, respectively, as compared to the reference NC_039217 genome) have been deposited in the GenBank database under accession numbers OQ750683 (PV1) and OQ750684 (PV2).
Plant samples
The leaf samples of 15 common bean plants of different origin grown in open field conditions in three locations in western Slovakia (Pezinok, Piešťany, Vrbová nad Váhom, Table 1) were harvested during vegetation period (July–August 2022) and tested for the PvEV-1 presence using RT-PCR (described below).
Additionally, the seeds of 20 bean genotypes of Slovak breeding origin (Table 2) long-term maintained in the curated seed collection of Slovak Republic germplasm collection in Piešťany were experimentally sown in a sterile garden soil and grown in an insect-proof cultivation room under controlled conditions (14 h light/10 h dark photoperiod, day/night temperature: 25/18°C). The same procedure was applied for the genotype Maxidor (of French origin), maintained in the international collection of bean varieties kept in the same germplasm collection. From each genotype, 4–6 seeds were sown and plantlets at the 4-5-true leaves stage were tested by RT-PCR using the apical leaves for total RNA isolation as described below.
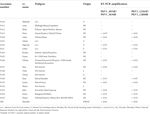
TABLE 2. Characteristics of the bean accessions from the Slovak bean germplasm collection tested by RT-PCR for PvEV-1 infection. The pedigree information was obtained from the genetic resources information system of Slovakia.1
RT-PCR and sequence analyses
Total RNAs were extracted from bean leaves using the Spectrum Plant Total RNA Kit (Sigma Aldrich, St. Louis, MO, United States). Subsequently, a two-step RT-PCR protocol was used. The first-strand cDNA was synthesized using random hexamer primers and the Avian myeloblastosis virus (AMV) reverse transcriptase (both from Promega Corp., Madison, WI, United States). For subsequent PCR amplifications, two different primer pairs targeting different genomic regions were used. A 703 bp long fragment partially spanning the viral helicase region was amplified using primers PhV1_4934F (5′- GATATGAGCAGTTCACCAGG—3′, forward) and PhV1_5636R (5′- CACCTTGTTGCTCCAAATCC—3′, reverse), corresponding to nucleotides 4,934 to 5,636 of the full-length sequence of the reference PvEV-1-Brazil isolate (NC_039217). The second primer pair PhV1_12265F (5′- CCAACCAGTTATAGACCATG—3′, forward) and PhV1_12860R (5′- TTGCTCTCCAATCACTACGG—3′, reverse) amplifies a 596-bp fragment located upstream of the RNA polymerase region (genome positions 12265 to 12860 of NC_039217). PCRs were performed using the proofreading TaKaRa Ex Taq™ polymerase (Takara Bio, Shiga, Japan). For both primer pairs, PCR amplification was performed under the following cycling conditions: initial denaturation at 94°C for 5 min; 35 cycles of 94°C for 20 s, 56°C for 20 s, and 72°C for 30 s; final extension step at 72°C for 10 min. As a negative control, total RNAs from Nicotiana benthamiana or total RNAs from the PvEV-1-negative P. vulgaris, cv. Melinda were used. The RT-PCR products were gel purified using the Wizard SV Gel and PCR Clean-Up System (Promega Corp., Madison, WI, United States), and directly sequenced in both directions by priming the sequencing reactions with the same oligonucleotides as used for PCR amplification. As the partial nucleotide sequences obtained from field or seed collection samples do not differ from the PV1 and PV2 sequences, they were not deposited in Genbank.
Phylogenetic analyses and comparisons with publicly available PvEV-1 sequences2 were performed using the MEGA v.7 (Kumar et al., 2016) and DnaSP v.5 (Librado and Rozas, 2009) programs.
Results
Identification and characterization of PvEV-1 in P. vulgaris from Slovakia using HTS
During an effort to assess the presence of viral pathogens naturally infecting common bean in Slovakia, two plants of cv. Maxidor were analyzed by HTS. While the PV1 plant showed no clear symptomatology besides a mild yellow chlorosis, the PV2 plant exhibited suspicious virus-like symptoms (deformation of leaf lamina, Supplementary Figure S1). The analysis of the HTS sequence data obtained from these two samples (PV1, 4,332,630 reads of mean length 113.5 nucleotides and PV2, 3,933,326 reads of mean length 117.4 nucleotides) enabled to obtain in both cases a large contig (ca. 13.02 and 11.43 kb, respectively) corresponding to PvEV-1. The mapping at high stringency of individual reads against the PvEV-1 reference genome (NC_039217) allowed the reconstruction of the nearly complete genomes for both isolates. The obtained PV1 genome consists of 14,054 nucleotides (150,152 mapped reads, mean coverage ×1192.4) while the PV2 one is 14,065 nucleotides long and reconstructed from 124,377 reads (mean coverage ×1030). No other known or novel viral agent was identified using the reads from the PV1 and PV2 samples.
The nearly complete PV1 and PV2 genomes show 99.9% nucleotide identity and differ only at 4 nucleotide positions (G4825A, A9115T, C9925T and G13980A, numbered according to NC_039217), while the encoded polyprotein sequences (4,619 amino acids) are strictly identical. PV1 and PV2 nucleotide identity with the reference PvEV-1 genome (NC_039217) reaches 98.4%, while the closed relative to PV1 and PV2 as judged by BlastN analysis is the Spanish PvEV-1 isolate MF375892 which is surprisingly reported to have been determined from an Entoleuca sp. fungus (nucleotide identity reaching 99.59%). Interestingly, when deduced polyprotein amino acid sequences are aligned, the Entoleuca sp. isolate present two highly divergent amino acid stretches as compared to all other PvEV-1 isolates, including the PV1 and PV2 ones, i.e., “FVCRLLLEKK” instead of “LVCCMGMGLM” at positions 152—161 and “FFQAEDGIR-” instead of “Y(K/RN)LTEDNCSR” at positions 3,491–3,500. The first stretch is situated in a part of polyprotein that does not contain any conserved protein motif, while second is located just before a conserved glycosyltransferase motif. Surprisingly, these two divergent regions of the Entoleuca isolate polyprotein are also related at the nucleotide sequence level since they largely correspond to a 28 nt inverted repeat (TCGTATGCCGTCTTCTGCTTGAAAAAAA, positions 624–648 and 10,642–10,666 in the MF375892 genome).
A phylogenetic analysis based on the nearly complete genome sequences revealed that all PvEV-1 isolates, including the PV1 and PV2 ones, group a very tight clade, clearly separated from the Phaseolus vulgaris alphaendornavirus-2 (PvEV-2) and PvEV-3, two other endornaviruses identified from bean plants (Figure 1). This clustering is also supported by the very low intragroup diversity among the 15 available PvEV-1 genomes, which reaches only 1.6% (±0.0). As expected from the Blast analysis, the two Slovak isolates group together with the Spanish isolate from Entoleuca (MF375892), with 100% bootstrap support.
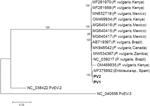
FIGURE 1. Phylogenetic tree generated from PvEV-1 nearly complete genome sequences. The phylogenetic tree was inferred using maximum likelihood (ML) and the General Time Reversible (GTR) model selected as the best-fit model of nucleotide substitution based on Bayesian information criterion (BIC) as implemented in MEGA 7. Isolates are identified by their GenBank accession numbers, host and country of origin. The Slovak isolates PV1 and PV2 sequenced during the present study are highlighted in bold. The genomes of the reference PvEV-2 and PvEV-3 isolates were also included. The scale bar indicates a genetic distance of 0.2. Bootstrap values higher than 70% (1,000 bootstrap resamplings) are indicated.
Confirmation by RT-PCR amplification of the presence of PvEV-1 in Slovakia
The initial identification of PvEV-1 in two bean plants during 2021 prompted efforts to screen additional bean plants for the virus presence. To this purpose, two primer pairs (PhV1_4934F/PhV1_5636R and PhV1_12265F/PhV1_12860R) were designed from a multiple alignment of 2 Slovak and 13 Genbank sequences. The two primer pairs were first validated using as template the PV1 and PV2 total RNAs used from the HTS experiment. In both cases, amplicons of the expected size (703 and 596 bp, respectively) were obtained and their direct sequencing confirmed the specificity of the amplification assay, confirming the presence of PvEV-1 in the analysed bean plants.
In a second step, a total of 15 leaf samples were obtained from open field-grown bean plants from three different locations, all sampled plants being asymptomatic (Table 1). Based on RT-PCR results, 4 out of 15 samples tested positive for PvEV-1, with identical results obtained with both primer pairs. Although two of these PvEV-1-positive samples were from cv. Maxidor and obtained from the same private garden in Pezinok, these two plants came from two different commercial seed lots originating from different suppliers. Direct dideoxy sequencing of PhV1_4934F/PhV1_5636R and PhV1_12265F/PhV1_12860R amplicons showed that the four amplified isolates were 100% identical with the PV1 and PV2 isolates in the two amplified regions.
Detection of PvEV-1 in the curated Slovak bean germplasm collection
In order to evaluate the presence of PvEV-1 in the Slovak Republic bean germplasm collection, the seeds of 20 Slovak and 1 French genotype in long-term storage were sown and the resulting plantlets individually tested by RT-PCR. No symptoms were observed in any of the analysed plants. Based on the RT-PCR results, 12 out of 21 genotypes tested PvEV-1-positive. Eleven of the positive genotypes originated from the Slovak breeding program (Petra, Anka, Nigrona, Lolita, Ultima, Sina, Ema, Lucka, Julia, Greta, Diana) and one genotype was of French origin (Maxidor). In most cases, the testing of several plants of the same genotype (4–6 seeds) provided consistent results (100% infection). However, in the case of cv. Petra, Ultima and Julia, one of the tested plants tested PvEV-1-negative (Table 2).
Discussion
In the last decade, HTS has become an unprecedented tool for unbiased virome analysis and characterization (Villamor et al., 2019; Maclot et al., 2020). Most of the studies confirmed that plants can be simultaneously infected by several viruses, among which are also different endornaviruses (e.g., Okada et al., 2011; Tomašechová et al., 2020).
As part of an effort to analyse the virome of legumes, HTS revealed the presence of PvEV-1 in two common bean plants grown in western Slovakia. The two assembled PvEV-1 genomes showed 99.9% sequence identity. Although the PV2 plant showed symptoms resembling those caused by viruses (Supplementary Figure S1), no other virus than PvEV-1 was identified from the sequencing reads. However, the symptoms observed are most likely not caused by PvEV-1, which has never been reported to be pathogenic, but are rather physiological. Indeed, the symptoms disappeared at a later stage.
To our knowledge, the PV1 and PV2 isolates are the first European PvEV-1 isolates originating from common bean, since the third available European PvEV-1 sequence, from Spain (MF375892, Velasco et al., 2019) is surprisingly reported to have been obtained from a fungus, Entoleuca sp. Interestingly, the genome of this Entoleuca isolate contains two highly divergent stretches as compared to all other known PvEV-1 isolates, that impact the encoded polyprotein sequence in regions of unknown function. Although this variability is potentially associated with the host, the confirmation of PvEV-1 infection in Entoleuca and the analysis of further Entoleuca isolates would be needed to confirm a direct relationship between host and virus sequence in these two regions. Interestingly, despite the divergence of these short regions, MF375892 shows the highest nucleotide identity with the PV1 and PV2 isolates among all known PvEV-1 isolates originating from Africa and Central/South America, suggesting that geography rather than host might structure the genetic variability of PvEV-1. However, further characterization of PvEV-1 isolates from different host genotypes and geographic origin is clearly needed to better understand the structuring of PvEV-1 genetic diversity.
The initial HTS-based identification of PvEV-1 prompted subsequent analyses to reveal PvEV-1 presence in Slovakia. These efforts focused on two groups of samples, i.e., field-grown bean plants and bean genotypes from the Slovak bean germplasm collection. In both cases, the presence of PvEV-1 infected plants was detected using two newly designed primer pairs targeting two different genome regions. Both primer pairs were designed to target genomic regions conserved between the PV-1 and PV-2 isolates as well as all 13 other PvEV-1 sequences present in GenBank. Interestingly, the sequencing of RT-PCR amplicons revealed that similar to GenBank sequences, all the newly detected Slovak isolates are identical in the two amplified genomic regions, further confirming the low molecular heterogeneity between PvEV-1 isolates possibly due to a high selection pressure imposed to PvEV-1 populations undergoing the vertical transmission (Butkovic and Gonzalez, 2022).
It is commonly accepted that endornaviruses rely on a kind of neutral or symbiotic relationship with their host, being harmlessly present within the cells in a persistent manner (Roossinck, 2010). Due to the vertical transmission, they are postulated to have very long evolutionary relationships with their hosts. Bean of cv. Maxidor (a French bean cultivar with a bushy growth) has been tested PvEV-1-positive irrespective of the year of sampling or supplier (Tables 1, 2), suggesting the virus persistence and an apparently efficient way of coevolution and vertical transmission. Endornaviruses have thus the potential to be used as clues in the reconstruction of pedigrees or of plant species evolutionary history at larger scales (Khankhum et al., 2015).
Overall, our results suggest a relatively high incidence of PvEV-1 in P. vulgaris in Slovakia, which is in line with other studies from different non-European geographical regions (Khankhum et al., 2015; Mutuku et al., 2018; Okada et al., 2018). Especially, the PvEV-1 presence was detected in plants germinated from seeds of more than a half of P. vulgaris genotypes maintained in the curated Slovak Gene Bank collection, extending the data on its common bean host range.
Data availability statement
The datasets presented in this study can be found in online repositories. The names of the repository/repositories and accession number(s) can be found below: https://www.ncbi.nlm.nih.gov/genbank/, OQ750683, OQ750684.
Author contributions
Conceptualization, MM and MG; methodology, MM, AA, PA, and MG; investigation, MM, AA, PA, LP, ZŠ, KŠ, and MG; resources, MM, LP, EZ, PH, and MG; data curation, AA, PA, KŠ, TC, and MG; writing -original draft preparation, TC and MG; writing -review and editing, MM, AA, PA, LP, ZŠ, EZ, PH, KŠ, TC, and MG; funding acquisition, MG. All authors contributed to the article and approved the submitted version.
Funding
This research was supported by grant APVV-20-0015 from the Slovak Research and Development Agency and VEGA 2/0030/20 from the Scientific Grant Agency of the Ministry of Education and Slovak Academy of Sciences. This publication was created thanks to support under the Operational Programme Integrated Infrastructure for the project: Strengthening of Research, Development, and Innovation Capacities of Translational Biomedical Research of Human Diseases, IMTS: 313021BZC9, co-financed by the European Regional Development Fund.
Conflict of interest
The authors declare that the research was conducted in the absence of any commercial or financial relationships that could be construed as a potential conflict of interest.
Supplementary material
The Supplementary Material for this article can be found online at: https://www.frontierspartnerships.org/articles/10.3389/av.2023.11484/full#supplementary-material
Footnotes
2accessed from https://www.ncbi.nlm.nih.gov/nuccore/ on 1st February 2023
References
Butkovic, A., and Gonzalez, R. (2022). A brief view of factors that affect plant virus evolution. Front. Virol. 2, 994057. doi:10.3389/fviro.2022.994057
Candresse, T., Marais, A., Sorrentino, R., Faure, C., Theil, S., Cadot, V., et al. (2016). Complete genomic sequence of barley (Hordeum vulgare) endornavirus (HvEV) determined by next-generation sequencing. Arch. Virol. 161, 741–743. doi:10.1007/s00705-015-2709-3
Fukuhara, T. (2019). Endornaviruses: Persistent dsRNA viruses with symbiotic properties in diverse eukaryotes. Virus Genes 55, 165–173. doi:10.1007/s11262-019-01635-5
Khankhum, S., Valverde, R. A., Pastor-Corrales, M. A., Osorno, J. M., and Sabanadzovic, S. (2015). Two endornaviruses show differential infection patterns between gene pools of Phaseolus vulgaris. Arch. Virol. 160, 1131–1137. doi:10.1007/s00705-015-2335-0
Kumar, S., Stecher, G., and Tamura, K. (2016). MEGA7: Molecular evolutionary genetics analysis version 7.0 for bigger datasets. Mol. Biol. Evol. 33, 1870–1874. doi:10.1093/molbev/msw054
Lefebvre, A., Scalla, R., and Pfeiffer, P. (1990). The double-stranded RNA associated with the '447' cytoplasmic male sterility in Vicia faba is packaged together with its replicase in cytoplasmic membranous vesicles. Plant Mol. Biol. 14, 477–490. doi:10.1007/BF00027494
Li, W., Zhang, H., Shu, Y., Cao, S., Sun, H., Zhang, A., et al. (2021). Genome structure and diversity of novel endornaviruses from wheat sharp eyespot pathogen Rhizoctonia cerealis. Virus Res. 297, 198368. doi:10.1016/j.virusres.2021.198368
Librado, P., and Rozas, J. (2009). DnaSP v5: A software for comprehensive analysis of DNA polymorphism data. Bioinformatics 25, 1451–1452. doi:10.1093/bioinformatics/btp187
Luo, X., Jiang, D., Xie, J., Jia, J., Duan, J., Cheng, J., et al. (2022). Genome characterization and phylogenetic analysis of a novel endornavirus that infects fungal pathogen Sclerotinia sclerotiorum. Viruses 14, 456. doi:10.3390/v14030456
Maclot, F., Candresse, T., Filloux, D., Malmstrom, C. M., Roumagnac, P., van der Vlugt, R., et al. (2020). Illuminating an ecological blackbox: Using high throughput sequencing to characterize the plant virome across scales. Front. Microbiol. 11, 578064. doi:10.3389/fmicb.2020.578064
Malmstrom, C. M., Melcher, U., and Bosque-Pérez, N. A. (2011). The expanding field of plant virus ecology: Historical foundations, knowledge gaps, and research directions. Virus Res. 159, 84–94. doi:10.1016/j.virusres.2011.05.010
Mutuku, J. M., Wamonje, F. O., Mukeshimana, G., Njuguna, J., Wamalwa, M., Choi, S-K., et al. (2018). Metagenomic analysis of plant virus occurrence in common bean (Phaseolus vulgaris) in Central Kenya. Front. Microbiol. 9, 2939. doi:10.3389/fmicb.2018.02939
Nordenstedt, N., Marcenaro, D., Chilagane, D., Mwaipopo, B., Rajamaeki, M-L., Nchimbi-Msolla, S., et al. (2017). Pathogenic seedborne viruses are rare but Phaseolus vulgaris endornaviruses are common in bean varieties grown in Nicaragua and Tanzania. PLoS ONE 12, e0178242. doi:10.1371/journal.pone.0178242
Okada, R., Kiyota, E., Sabanadzovic, S., Moriyama, H., Fukuhara, T., Saha, P., et al. (2011). Bell pepper endornavirus: Molecular and biological properties, and occurrence in the genus Capsicum. J. Gen. Virol. 92, 2664–2673. doi:10.1099/vir.0.034686-0
Okada, R., Yong, C. K., Valverde, R. A., Sabanadzovic, S., Aoki, N., Hotate, S., et al. (2013). Molecular characterization of two evolutionarily distinct endornaviruses co-infecting common bean (Phaseolus vulgaris). J. Gen. Virol. 94, 220–229. doi:10.1099/vir.0.044487-0
Okada, R., Alcalá-Briseno, R. I., Escalante, C., Sabanadzovic, S., and Valverde, R. A. (2018). Genomic sequence of a novel endornavirus from Phaseolus vulgaris and occurrence in mixed infections with two other endornaviruses. Virus Res. 257, 63–67. doi:10.1016/j.virusres.2018.09.005
Osaki, H., Nakamura, H., Sasaki, A., Matsumoto, N., and Yoshida, K. (2006). An endornavirus from a hypovirulent strain of the violet root rot fungus, Helicobasidium mompa. Virus Res. 118, 143–149. doi:10.1016/j.virusres.2005.12.004
Pfeiffer, P., Jung, J. L., Heitzler, J., and Keith, G. (1993). Unusual structure of the double-stranded RNA associated with the '447' cytoplasmic male sterility in Vicia faba. J. Gen. Virol. 74, 1167–1173. doi:10.1099/0022-1317-74-6-1167
Pfeiffer, P. (1998). Nucleotide sequence, genetic organization and expression strategy of the double-stranded RNA associated with the '447' cytoplasmic male sterility trait in Vicia faba. J. Gen. Virol. 79, 2349–2358. doi:10.1099/0022-1317-79-10-2349
Roossinck, M. J., Sabanadzovic, S., Okada, R., and Valverde, R. A. (2011). The remarkable evolutionary history of endornaviruses. J. Gen. Virol. 92, 2674–2678. doi:10.1099/vir.0.034702-0
Roossinck, M. J. (2010). Lifestyles of plant viruses. Philos. Trans. R. Soc. Lond B Biol. Sci. 365, 1899–1905. doi:10.1098/rstb.2010.0057
Roossinck, M. J. (2015). A new look at plant viruses and their potential beneficial roles in crops. Mol. Plant Pathol. 16, 331–333. doi:10.1111/mpp.12241
Sabanadzovic, S., Wintermantel, W. M., Valverde, R. A., McCreight, J. D., and Aboughanem-Sabanadzovic, N. (2016). Cucumis melo endornavirus: Genome organization, host range and co-divergence with the host. Virus Res. 214, 49–58. doi:10.1016/j.virusres.2016.01.001
Takahashi, H., Fukuhara, T., Kitazawa, H., and Kormelink, R. (2019). Virus latency and the impact on plants. Front. Microbiol. 10, 2764. doi:10.3389/fmicb.2019.02764
Tomašechová, J., Hančinský, R., Predajňa, L., Kraic, J., Mihálik, D., Šoltys, K., et al. (2019). High-throughput sequencing reveals bell pepper endornavirus infection in pepper (Capsicum annum) in Slovakia and enables its further molecular characterization. Plants 9, 41. doi:10.3390/plants9010041
Valverde, R. A., Khalifa, M. E., Okada, R., Fukuhara, T., and Sabanadzovic, S., ICTV Report Consortium (2019). ICTV virus taxonomy profile: Endornaviridae. J. Gen. Virol. 100, 1204–1205. doi:10.1099/jgv.0.001277
Velasco, L., Arjona-Girona, I., Cretazzo, E., and López-Herrera, C. (2019). Viromes in Xylariaceae fungi infecting avocado in Spain. Virology 532, 11–21. doi:10.1016/j.virol.2019.03.021
Villamor, D. E. V., Ho, T., Al Rwahnih, M., Martin, R. R., and Tzanetakis, I. E. (2019). High throughput sequencing for plant virus detection and discovery. Phytopathology 109, 716–725. doi:10.1094/PHYTO-07-18-0257-RVW
Wakarchuk, D. A., and Hamilton, R. I. (1990). Partial nucleotide sequence from enigmatic dsRNAs in Phaseolus vulgaris. Plant Mol. Biol. 14, 637–639. doi:10.1007/bf00027512
Wang, J., Ni, Y., Liu, X., Zhao, H., Xiao, Y., Xiao, X., et al. (2020). Divergent RNA viruses in Macrophomina phaseolina exhibit potential as virocontrol agents. Virus Evol. 7, veaa095. doi:10.1093/ve/veaa095
Yang, D., Wu, M., Zhang, J., Chen, W., Li, G., and Yang, L. (2018). Sclerotinia minor endornavirus 1, a novel pathogenicity debilitation-associated mycovirus with a wide spectrum of horizontal transmissibility. Viruses 10, 589. doi:10.3390/v10110589
Keywords: bean, Endornaviridae, genotype, PvEV-1, vertical transmission
Citation: Mrkvová M, Achs A, Alaxin P, Šubr Z, Predajňa L, Zetochová E, Hauptvogel P, Šoltys K, Candresse T and Glasa M (2023) Phaseolus vulgaris alphaendornavirus-1 is frequent in bean germplasm in Slovakia and shows low molecular variability. Acta Virol. 67:11484. doi: 10.3389/av.2023.11484
Received: 17 April 2023; Accepted: 18 May 2023;
Published: 05 June 2023.
Edited by:
Katarina Polcicova, Slovak Academy of Sciences, SlovakiaReviewed by:
Petr Komínek, Crop Research Institute (CRI), CzechiaEva Varallyay, Hungarian University of Agricultural and Life Sciences, Hungary
Copyright © 2023 Mrkvová, Achs, Alaxin, Šubr, Predajňa, Zetochová, Hauptvogel, Šoltys, Candresse and Glasa. This is an open-access article distributed under the terms of the Creative Commons Attribution License (CC BY). The use, distribution or reproduction in other forums is permitted, provided the original author(s) and the copyright owner(s) are credited and that the original publication in this journal is cited, in accordance with accepted academic practice. No use, distribution or reproduction is permitted which does not comply with these terms.
*Correspondence: Miroslav Glasa, miroslav.glasa@savba.sk
†These authors have contributed equally to this work